How to optimize human biology
This article is part of the World Economic Forum's Geostrategy platform
The term ‘genome editing’ has reemerged as a hot topic in the last five years. Recent breakthroughs in the gene editing technology CRISPR have invigorated the biotechnology community with the promise of precisely manipulating the genome of any organism, including humans.
While many scientists express interest in this technology for basic research, the potential impact of genome editing in medical treatments looms in the distance. Decisions about the clinical use of CRISPR are coming, argues the Wilson Center report, How to Optimize Human Biology: Where Genome Editing and Artificial Intelligence Collide.
Knowledge of clustered regularly interspaced short palindromic repeats (CRISPR) arose from years of initial research on bacterial immunity, but experts have begun to recognize the power and potential of this technology in broader gene editing. The new method found in CRISPR offers significant advantages over existing methods like zinc finger nuclease (ZFN) and transcription activator-like effector nucleases (TALENs).
Experts now tout CRISPR as more efficient, simpler to use, and more able to edit many genes at once than older methods. Interest in this technology has grown exponentially in the last five years since scientists first demonstrated it could successfully edit DNA alone as well as DNA inside cells.
Cut and paste
CRISPR edits DNA by acting as a pair of “molecular scissors” to ‘cut’ and ‘paste’ DNA in the genome.
To accomplish this, the CRISPR-associated (Cas) protein uses a short segment of RNA to find the genomics sequence of interest.
This feature makes this technology easy to use, as experts only need to change the guide RNA to locate different genes and can utilize the same CRISPR-Cas unit. Once the CRISPR-Cas system arrives at the target gene, the Cas unit can edit the DNA using a variety of mechanisms to insert, delete, or replace DNA at that site. This function should prevent CRISPR-Cas from making edits on other genes, as it should only edit once it arrives at the target. The variety of genome editing functions this technology can perform also contribute to its wide applicability.
Medicine for the Next Generation(s)
Some of the most significant applications of genome editing include medical uses for humans, as CRISPR could be deployed to repair disease-causing mutations.
Practitioners could deploy this technology in the clinic in two broad ways, somatically or in germline modifications.
Somatic therapy refers to editing the DNA in the cells of a human after birth, rather than during prenatal development. New types of therapy for existing diseases like cancer could appear when utilizing this technology, including editing immune cells to better target cancer.
This application of CRISPR represents another kind of gene therapy, which is not unprecedented and presents less controversy than other uses of the technology. CRISPR as gene therapy may appear in clinics in the short-to-medium term, especially as clinical trials may begin soon in the United States, and many more have already begun or will start soon in China.
Both the greater controversy and potential around CRISPR comes from the future potential to perform germline genomic editing. This form of therapy could prevent genetic risk or disease before it occurs by editing the genome of an egg, sperm, or embryo and then using in vitro fertilization to develop a child.
Editing out cancer
Rather than simply treating cancer as it arises in adults, CRISPR promises the ability to edit out mutations from genes such as BRCA1 to lower the cancer risk of children even before birth.
Genome editing similarly could correct mutations which cause debilitating genetic conditions including Huntington’s disease or cystic fibrosis, ensuring future generations will not suffer from such ailments.
However, techniques for germline editing pose safety and ethical issues both for the child born and for any children they have, as these genetic modifications will appear in subsequent generations.
Off-target effects, or the possibility of altering unintended parts of the genome, have presented an increased concern since a publication in Nature Methods suggested such effects occur more often than previously thought.
Germline CRISPR use can result in mosaicism, where successful genome edits occurs in only a fraction of an embryo’s cells. Such non-uniform edits may fail to prevent a disease from occurring or could pose novel health complications.
A recent development in methods involving earlier exposure of an embryo to CRISPR may aid in reducing off-target effects and mosaicism (see illustration below), though these groundbreaking findings will benefit from further study.
Epigenetic effects may further complicate germline editing, as epigenetic factors augment an organism’s genome after conception to impact whether, where, and how the body expresses genes.
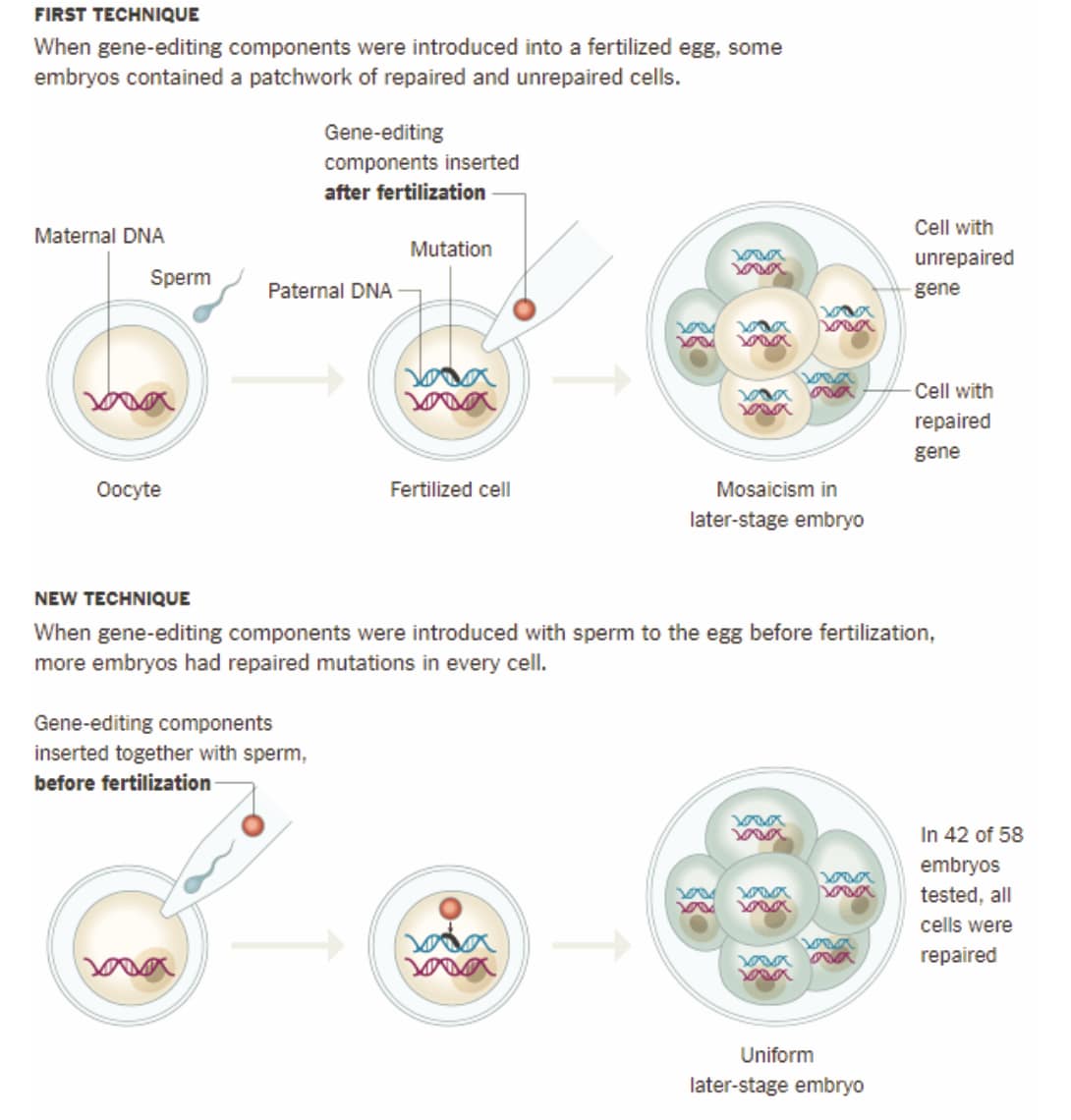
Somatic therapies may have effectiveness problems as well, as the human immune system could potentially (and correctly) recognize the CRISR-Cas9 enzyme as non-human and develop antibodies that inhibit these gene editing tools.
Medical use of CRISPR germline editing would almost certainly necessitate Congressional action. Current appropriations forbid the National Institutes of Health (NIH) from funding research which creates or destroys human embryos and provide no funding for the Food and Drug Administration (FDA) to review germline editing products.
These barriers functionally prevent medical research on human germline editing in the US. While clinical use will require the above issues to be resolved, it remains likely that CRISPR germline editing may appear as clinically available in the medium-to-long term.
Biology as a Machine
The advent of molecular biology, genomics, and now CRISPR have promoted a conceptualization of biology as a machine. This view describes cellular and genomic functions using comparisons to engineering or software concepts.
Scientists describe mitochondria as “powerhouses,” kinesins as “motors” that move “cargo,” and insulin producing microorganisms as “factories.” Understanding natural and synthetic biology in these terms allows for simplified communication of complex ideas without steeping the discourse in field-specific jargon. This presents benefits for scientists intending on communicating with others outside of their field, the public, or decision makers.
However, expressing biological concepts primarily with engineering analogies may have second-order effects on how the researchers view their work and how these fields advance.
Viewing biology as a machine arises from the core philosophy of synthetic biology – the engineering concept of design applied to assembling new biological systems to perform a task.
Practitioners conceptualize building new organisms by using living ‘building blocks’ and genetically ‘programming’ the living machine to perform a wide spectrum of tasks.
This programmability of genomes in living machines allows for synthetic biologists to design novel functions into their biological machines, which may not appear in nature, and gives way to a problem solving mentality towards living systems.
Scientists have similarly embarked on work to utilize DNA for data storage, with a group succeeding in storing a movie and computer operating system in DNA. This exposition of biology into computer science reflects a larger shift of conceptualizing biology as a machine, which can be programmed and designed to perform tasks, as any other machine.
Artificial intelligence could revolutionize genomics
Artificial intelligence (AI) represents an emerging technology with the potential to significantly impact the medical field. Progress in deep learning has enabled this shift, in which AI can learn by experience. This type of learning bears similarities to how children acquire new information, and the technology functions by utilizing programme architecture that resembles the human brain.
Such an approach involves machine intelligence recognizing patterns in data and learning from its mistakes to better identify or classify new information. Deep learning specifically supports this function of AI by allowing the machine to recognize many layers of patterns; like identifying an animal by first recognizing its outline, then focusing on more specific details like fur.
This technology continues to find use in a variety of settings from recognizing cats in YouTube videos, to predicting RNA splicing patterns in mouse cells. AI technology can additionally utilize optimization tools and even contribute to new optimization methods. These capacities of deep learning to identify patterns from large datasets and optimize systems may provide a powerful tool when combined with genome editing.
Due to advances in AI, this technology holds new potential uses in the clinic and in medical research.
Deep learning
Deep learning software has demonstrated its ability to diagnose images of skin cancer and microscopy images, and predict disease in hospitals after reviewing patient medical records.
These AI learn to diagnose medical conditions by utilizing experiential knowledge, resembling how physicians are trained during residency.
Beyond the clinic, the technology can provide powerful analyses of currently available scientific information to model the molecular mechanics of how genetic variation causes disease.
Similarly, AI appears set to analyze a large number of genetic datasets, in a partnership between Google and Genomic England. This capacity of deep learning to diagnose, predict, and find new patterns in how genetic disease operates could make AI an invaluable companion to clinical medicine. Access to cloud computing stands to further bolster these abilities as well as patient accessibility.
As AI continues to expand into the medical realm, the tool could have a powerful effect on how practitioners utilize genome editing. Since the new biology aims to optimize living machines to best perform their intended functions, gene-editing practitioners may take this mentality to maximizing a patient’s ability to live a healthy life. Particularly if human biology is viewed as a machine, deep learning could find use in instructing experts on where to use technologies like CRISPR in the genome to ‘optimize’ the human body.
Don't miss any update on this topic
Create a free account and access your personalized content collection with our latest publications and analyses.
License and Republishing
World Economic Forum articles may be republished in accordance with the Creative Commons Attribution-NonCommercial-NoDerivatives 4.0 International Public License, and in accordance with our Terms of Use.
The views expressed in this article are those of the author alone and not the World Economic Forum.
Forum Stories newsletter
Bringing you weekly curated insights and analysis on the global issues that matter.