How could discarded tree forks help tackle climate change?
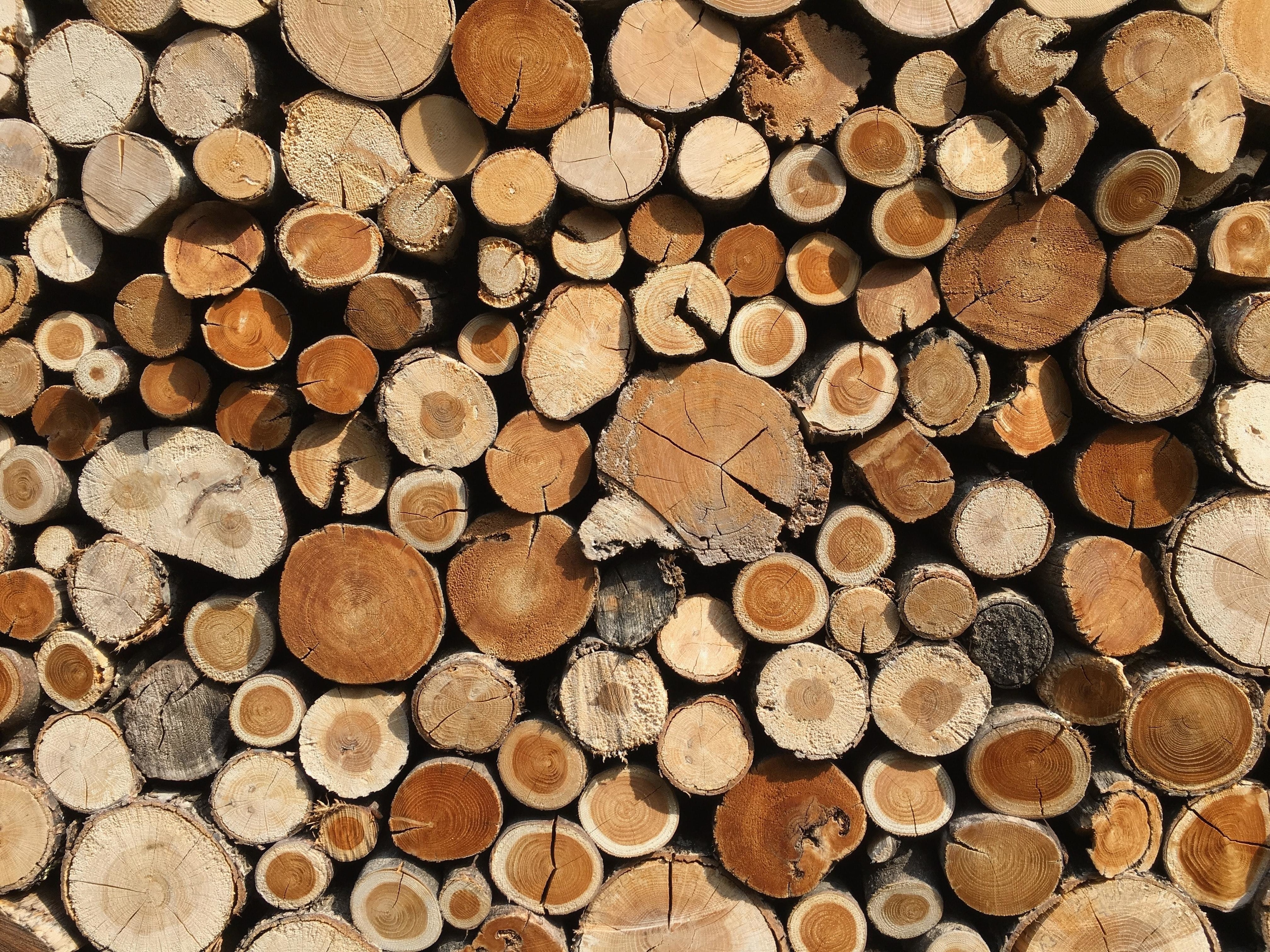
Wood provides a natural form of carbon sequestration. Image: UNSPLASH/ Bill Johnston
- With the concrete and steel industries responsible for 15% of global carbon dioxide emissions, there are increasing calls for the use of timber as a more sustainable construction material.
- Professor Caitlin Mueller has developed a five-step “design-to-fabrication workflow” that takes the structure of wood and combines it with digital architectural tools.
- A tree fork provides a natural example of a strong internal structure that can be replicated in architectural form.
Concern about climate change has focused significant attention on the buildings sector, in particular on the extraction and processing of construction materials. The concrete and steel industries together are responsible for as much as 15 percent of global carbon dioxide emissions. In contrast, wood provides a natural form of carbon sequestration, so there’s a move to use timber instead. Indeed, some countries are calling for public buildings to be made at least partly from timber, and large-scale timber buildings have been appearing around the world.
Observing those trends, Caitlin Mueller ’07, SM ’14, PhD ’14, an associate professor of architecture and of civil and environmental engineering in the Building Technology Program at MIT, sees an opportunity for further sustainability gains. As the timber industry seeks to produce wooden replacements for traditional concrete and steel elements, the focus is on harvesting the straight sections of trees. Irregular sections such as knots and forks are turned into pellets and burned, or ground up to make garden mulch, which will decompose within a few years; both approaches release the carbon trapped in the wood to the atmosphere.
For the past four years, Mueller and her Digital Structures research group have been developing a strategy for “upcycling” those waste materials by using them in construction — not as cladding or finishes aimed at improving appearance, but as structural components. “The greatest value you can give to a material is to give it a load-bearing role in a structure,” she says. But when builders use virgin materials, those structural components are the most emissions-intensive parts of buildings due to their large volume of high-strength materials. Using upcycled materials in place of those high-carbon systems is therefore especially impactful in reducing emissions.
Mueller and her team focus on tree forks — that is, spots where the trunk or branch of a tree divides in two, forming a Y-shaped piece. In architectural drawings, there are many similar Y-shaped nodes where straight elements come together. In such cases, those units must be strong enough to support critical loads.
“Tree forks are naturally engineered structural connections that work as cantilevers in trees, which means that they have the potential to transfer force very efficiently thanks to their internal fiber structure,” says Mueller. “If you take a tree fork and slice it down the middle, you see an unbelievable network of fibers that are intertwining to create these often three-dimensional load transfer points in a tree. We’re starting to do the same thing using 3D printing, but we’re nowhere near what nature does in terms of complex fiber orientation and geometry.”
She and her team have developed a five-step “design-to-fabrication workflow” that combines natural structures such as tree forks with the digital and computational tools now used in architectural design. While there’s long been a “craft” movement to use natural wood in railings and decorative features, the use of computational tools makes it possible to use wood in structural roles — without excessive cutting, which is costly and may compromise the natural geometry and internal grain structure of the wood.
Given the wide use of digital tools by today’s architects, Mueller believes that her approach is “at least potentially scalable and potentially achievable within our industrialized materials processing systems.” In addition, by combining tree forks with digital design tools, the novel approach can also support the trend among architects to explore new forms. “Many iconic buildings built in the past two decades have unexpected shapes,” says Mueller. “Tree branches have a very specific geometry that sometimes lends itself to an irregular or nonstandard architectural form — driven not by some arbitrary algorithm but by the material itself.”
Step 0: Find a source, set goals
Before starting their design-to-fabrication process, the researchers needed to locate a source of tree forks. Mueller found help in the Urban Forestry Division of the City of Somerville, Massachusetts, which maintains a digital inventory of more than 2,000 street trees — including more than 20 species — and records information about the location, approximate trunk diameter, and condition of each tree.
With permission from the forestry division, the team was on hand in 2018 when a large group of trees was cut down near the site of the new Somerville High School. Among the heavy equipment on site was a chipper, poised to turn all the waste wood into mulch. Instead, the workers obligingly put the waste wood into the researchers’ truck to be brought to MIT.
In their project, the MIT team sought not only to upcycle that waste material but also to use it to create a structure that would be valued by the public. “Where I live, the city has had to take down a lot of trees due to damage from an invasive species of beetle,” Mueller explains. “People get really upset — understandably. Trees are an important part of the urban fabric, providing shade and beauty.” She and her team hoped to reduce that animosity by “reinstalling the removed trees in the form of a new functional structure that would recreate the atmosphere and spatial experience previously provided by the felled trees.”
With their source and goals identified, the researchers were ready to demonstrate the five steps in their design-to-fabrication workflow for making spatial structures using an inventory of tree forks.
Step 1: Create a digital material library
The first task was to turn their collection of tree forks into a digital library. They began by cutting off excess material to produce isolated tree forks. They then created a 3D scan of each fork. Mueller notes that as a result of recent progress in photogrammetry (measuring objects using photographs) and 3D scanning, they could create high-resolution digital representations of the individual tree forks with relatively inexpensive equipment, even using apps that run on a typical smartphone.
In the digital library, each fork is represented by a “skeletonized” version showing three straight bars coming together at a point. The relative geometry and orientation of the branches are of particular interest because they determine the internal fiber orientation that gives the component its strength.
Step 2: Find the best match between the initial design and the material library
Like a tree, a typical architectural design is filled with Y-shaped nodes where three straight elements meet up to support a critical load. The goal was therefore to match the tree forks in the material library with the nodes in a sample architectural design.
First, the researchers developed a “mismatch metric” for quantifying how well the geometries of a particular tree fork aligned with a given design node. “We’re trying to line up the straight elements in the structure with where the branches originally were in the tree,” explains Mueller. “That gives us the optimal orientation for load transfer and maximizes use of the inherent strength of the wood fiber.” The poorer the alignment, the higher the mismatch metric.
The goal was to get the best overall distribution of all the tree forks among the nodes in the target design. Therefore, the researchers needed to try different fork-to-node distributions and, for each distribution, add up the individual fork-to-node mismatch errors to generate an overall, or global, matching score. The distribution with the best matching score would produce the most structurally efficient use of the total tree fork inventory.
Since performing that process manually would take far too long to be practical, they turned to the “Hungarian algorithm,” a technique developed in 1955 for solving such problems. “The brilliance of the algorithm is solving that [matching] problem very quickly,” Mueller says. She notes that it’s a very general-use algorithm. “It’s used for things like marriage match-making. It can be used any time you have two collections of things that you’re trying to find unique matches between. So, we definitely didn’t invent the algorithm, but we were the first to identify that it could be used for this problem.”
The researchers performed repeated tests to show possible distributions of the tree forks in their inventory and found that the matching score improved as the number of forks available in the material library increased — up to a point. In general, the researchers concluded that the mismatch score was lowest, and thus best, when there were about three times as many forks in the material library as there were nodes in the target design.
Step 3: Balance designer intention with structural performance
The next step in the process was to incorporate the intention or preference of the designer. To permit that flexibility, each design includes a limited number of critical parameters, such as bar length and bending strain. Using those parameters, the designer can manually change the overall shape, or geometry, of the design or can use an algorithm that automatically changes, or “morphs,” the geometry. And every time the design geometry changes, the Hungarian algorithm recalculates the optimal fork-to-node matching.
“Because the Hungarian algorithm is extremely fast, all the morphing and the design updating can be really fluid,” notes Mueller. In addition, any change to a new geometry is followed by a structural analysis that checks the deflections, strain energy, and other performance measures of the structure. On occasion, the automatically generated design that yields the best matching score may deviate far from the designer’s initial intention. In such cases, an alternative solution can be found that satisfactorily balances the design intention with a low matching score.
Step 4: Automatically generate the machine code for fast cutting
When the structural geometry and distribution of tree forks have been finalized, it’s time to think about actually building the structure. To simplify assembly and maintenance, the researchers prepare the tree forks by recutting their end faces to better match adjoining straight timbers and cutting off any remaining bark to reduce susceptibility to rot and fire.
To guide that process, they developed a custom algorithm that automatically computes the cuts needed to make a given tree fork fit into its assigned node and to strip off the bark. The goal is to remove as little material as possible but also to avoid a complex, time-consuming machining process. “If we make too few cuts, we’ll cut off too much of the critical structural material. But we don’t want to make a million tiny cuts because it will take forever,” Mueller explains.
The team uses facilities at the Autodesk Boston Technology Center Build Space, where the robots are far larger than any at MIT and the processing is all automated. To prepare each tree fork, they mount it on a robotic arm that pushes the joint through a traditional band saw in different orientations, guided by computer-generated instructions. The robot also mills all the holes for the structural connections. “That’s helpful because it ensures that everything is aligned the way you expect it to be,” says Mueller.
Step 5: Assemble the available forks and linear elements to build the structure
The final step is to assemble the structure. The tree-fork-based joints are all irregular, and combining them with the precut, straight wooden elements could be difficult. However, they’re all labeled. “All the information for the geometry is embedded in the joint, so the assembly process is really low-tech,” says Mueller. “It’s like a child’s toy set. You just follow the instructions on the joints to put all the pieces together.”
They installed their final structure temporarily on the MIT campus, but Mueller notes that it was only a portion of the structure they plan to eventually build. “It had 12 nodes that we designed and fabricated using our process,” she says, adding that the team’s work was “a little interrupted by the pandemic.” As activity on campus resumes, the researchers plan to finish designing and building the complete structure, which will include about 40 nodes and will be installed as an outdoor pavilion on the site of the felled trees in Somerville.
In addition, they will continue their research. Plans include working with larger material libraries, some with multibranch forks, and replacing their 3D-scanning technique with computerized tomography scanning technologies that can automatically generate a detailed geometric representation of a tree fork, including its precise fiber orientation and density. And in a parallel project, they’ve been exploring using their process with other sources of materials, with one case study focusing on using material from a demolished wood-framed house to construct more than a dozen geodesic domes.
To Mueller, the work to date already provides new guidance for the architectural design process. With digital tools, it has become easy for architects to analyze the embodied carbon or future energy use of a design option. “Now we have a new metric of performance: How well am I using available resources?” she says. “With the Hungarian algorithm, we can compute that metric basically in real time, so we can work rapidly and creatively with that as another input to the design process.”
What is the World Economic Forum doing to promote sustainable urban development?
Don't miss any update on this topic
Create a free account and access your personalized content collection with our latest publications and analyses.
License and Republishing
World Economic Forum articles may be republished in accordance with the Creative Commons Attribution-NonCommercial-NoDerivatives 4.0 International Public License, and in accordance with our Terms of Use.
The views expressed in this article are those of the author alone and not the World Economic Forum.
Stay up to date:
Engineering and Construction
Forum Stories newsletter
Bringing you weekly curated insights and analysis on the global issues that matter.