5 things to know about carbon mineralization, a new approach to carbon removal
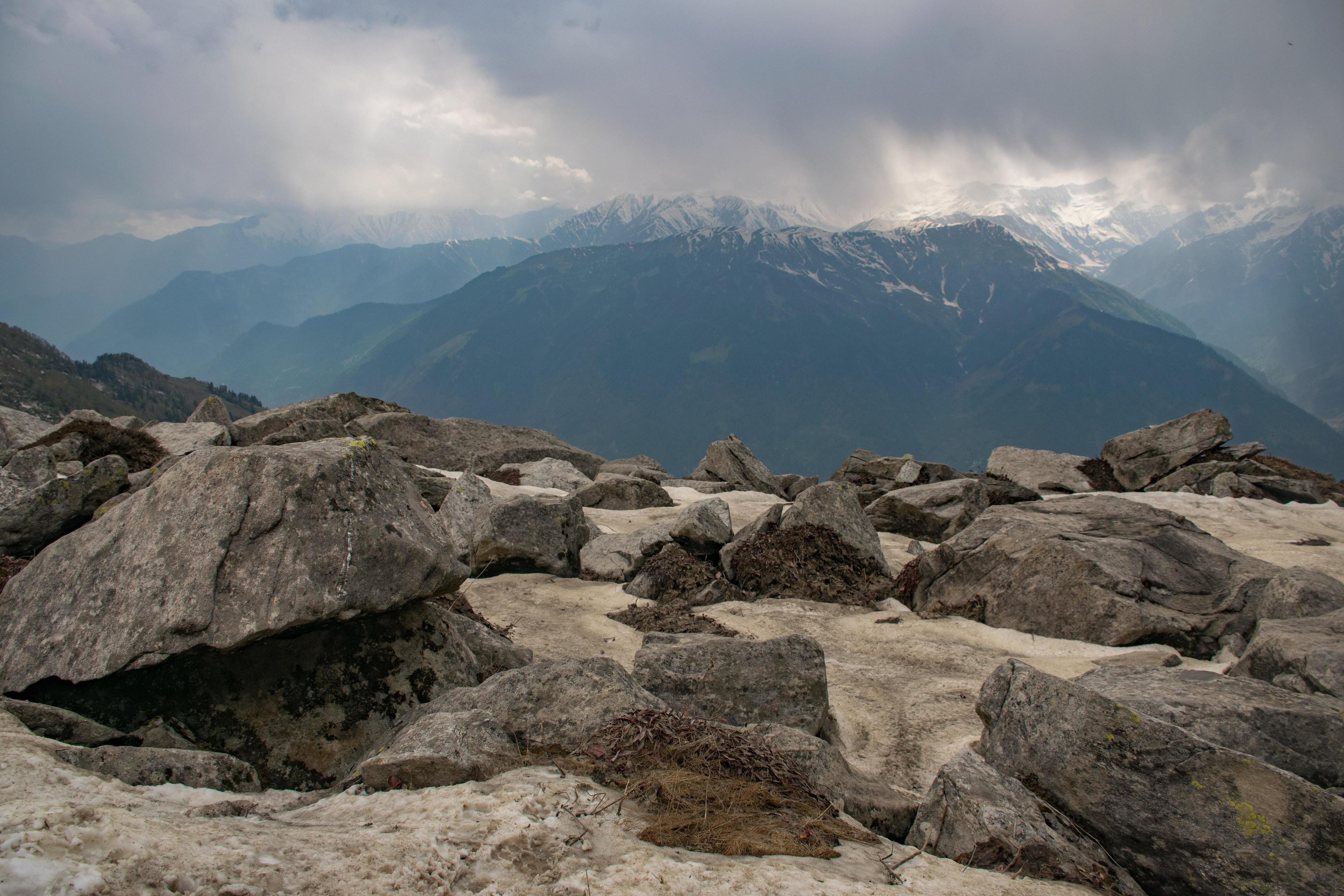
Carbon mineralization has the potential to remove significant amounts of CO2 from the atmosphere. Image: Pexels/Guduru Ajay Bhargav
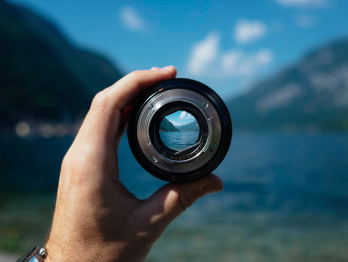
Get involved with our crowdsourced digital platform to deliver impact at scale
Stay up to date:
SDG 13: Climate Action
- Carbon mineralization is a process that accelerates the reaction between carbon dioxide (CO2) and certain minerals, removing CO2 from the atmosphere and permanently storing it.
- This process occurs naturally over hundreds or thousands of years, but it can be sped up in numerous ways to remove large amounts of atmospheric CO2 in the coming decades.
- Carbon mineralization has significant potential to remove CO2 from the atmosphere, but it has not yet been widely deployed.
- However, more research is needed to understand the environmental and social impacts of carbon mineralization.
Carbon mineralization is a carbon dioxide removal (CDR) approach that is beginning to attract interest as the world seeks to prevent some of the worst impacts of climate change. It accelerates reactions between carbon dioxide (CO2) and certain minerals, removing CO2 from the atmosphere and permanently storing it. Although this process occurs naturally over hundreds or thousands of years, carbon mineralization can be sped up in numerous ways to remove large amounts of atmospheric CO2 in the coming decades.
As global temperatures continue to rise, greenhouse gas emissions — especially CO2 — must be dramatically reduced to limit global warming to 1.5 degrees C (2.7 degrees F), as outlined in the international Paris Agreement on climate change. There is scientific consensus that along with deep emissions reductions, reaching these goals will also require us to remove CO2 already in the atmosphere. Although deep emission reductions must remain the highest priority, CDR approaches like carbon mineralization must complement these efforts.
Mineralization presents significant carbon removal potential but has lacked sufficient research and demonstration funding that could eventually bring it into wider use. More study and testing are needed to better understand how and where it could be most effective as well as potential environmental and social impacts.
Here are the answers to five common questions about carbon mineralization:
1) What is carbon mineralization?
Carbon mineralization is a process that naturally occurs over hundreds or thousands of years in which certain minerals inside rocks react with atmospheric CO2 to create carbonates, solid minerals that securely remove and sequester CO2.
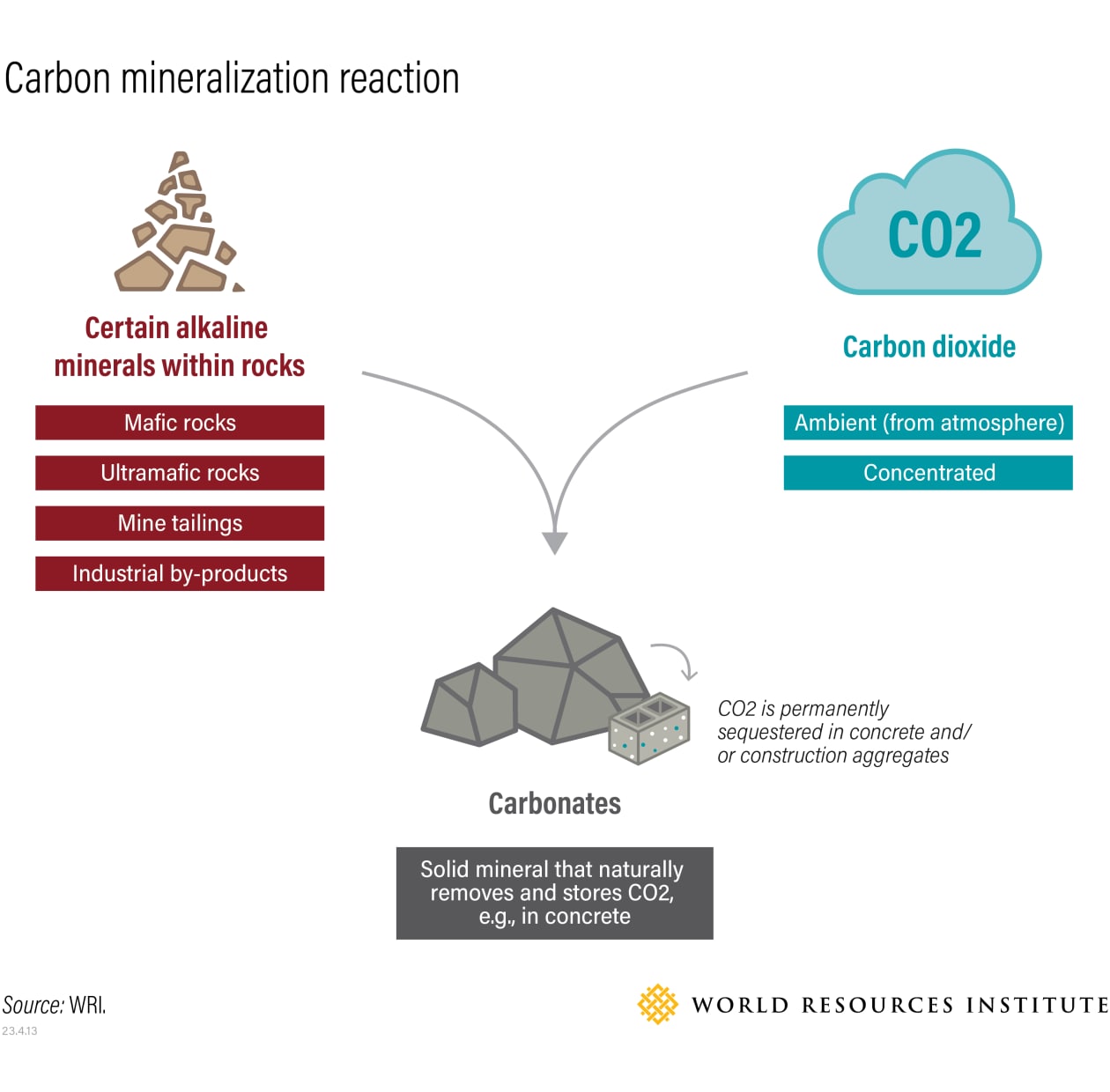
This chemical reaction works best with mafic or ultramafic rocks, which are highly reactive to CO2, as they contain alkaline minerals like magnesium or calcium-bearing silicates. Through these natural sink processes, carbon mineralization permanently removes about 0.3 billion metric tons (gigatons) of CO2 from the atmosphere each year, which is a little more than Poland’s annual emissions.
There are ways to accelerate this natural mineralization process, allowing for even more atmospheric CO2 to be removed and stored in the natural environment — or in products, such as concrete. Scientists estimate that up to 1 gigaton of CO2 per year could be removed from the atmosphere globally by 2035, and 10 gigatons of CO2 per year by 2050, if we invest sufficient funds in research and testing today to prepare for larger-scale deployment in the coming years. For reference, current annual global CO2 emissions are around 40 gigatons.
Accelerated mineralization processes can take anywhere from hours to years, depending on factors including mineral composition, temperature, humidity and which sources of CO2 and alkaline feedstocks are used in the approach.
2) What are the different carbon mineralization approaches?
Broadly speaking, carbon mineralization takes place either in the subsurface of the Earth (known as in-situ carbon mineralization) or at the Earth’s surface (known as ex-situ or surficial carbon mineralization).
Subsurface
There are several in-situ mineralization methods. One involves dissolving concentrated CO2 in water —almost like an industrial sized soda stream — and then injecting it through wells into mafic or ultramafic rocks, which are highly reactive with CO2. There, carbonate minerals form from the reaction, sequestering the CO2. The CO2-depleted water is then brought back to the Earth’s surface.
Another method involves injecting supercritical CO2 (CO2 compressed to a density similar to water) into basalt rocks. This method requires significantly less water for the injection, although much greater energy for compression.
Subsurface injection of CO2 has been well studied in sedimentary rock reservoirs, such as where depleted oil reservoirs are repurposed for CO2 storage, but not as much in basalts for the purpose of in-situ carbon mineralization. Two existing projects (Wallula in Washington state and CarbFix in Iceland) have already demonstrated that basalt reservoirs are promising for in-situ carbon mineralization.
Basalt, which is permeable and porous, holds much promise for carbon mineralization because it can store great quantities of CO2. Basalts are abundant on volcanic islands and in flood basalt provinces, such as the Columbia River in the Pacific Northwest.
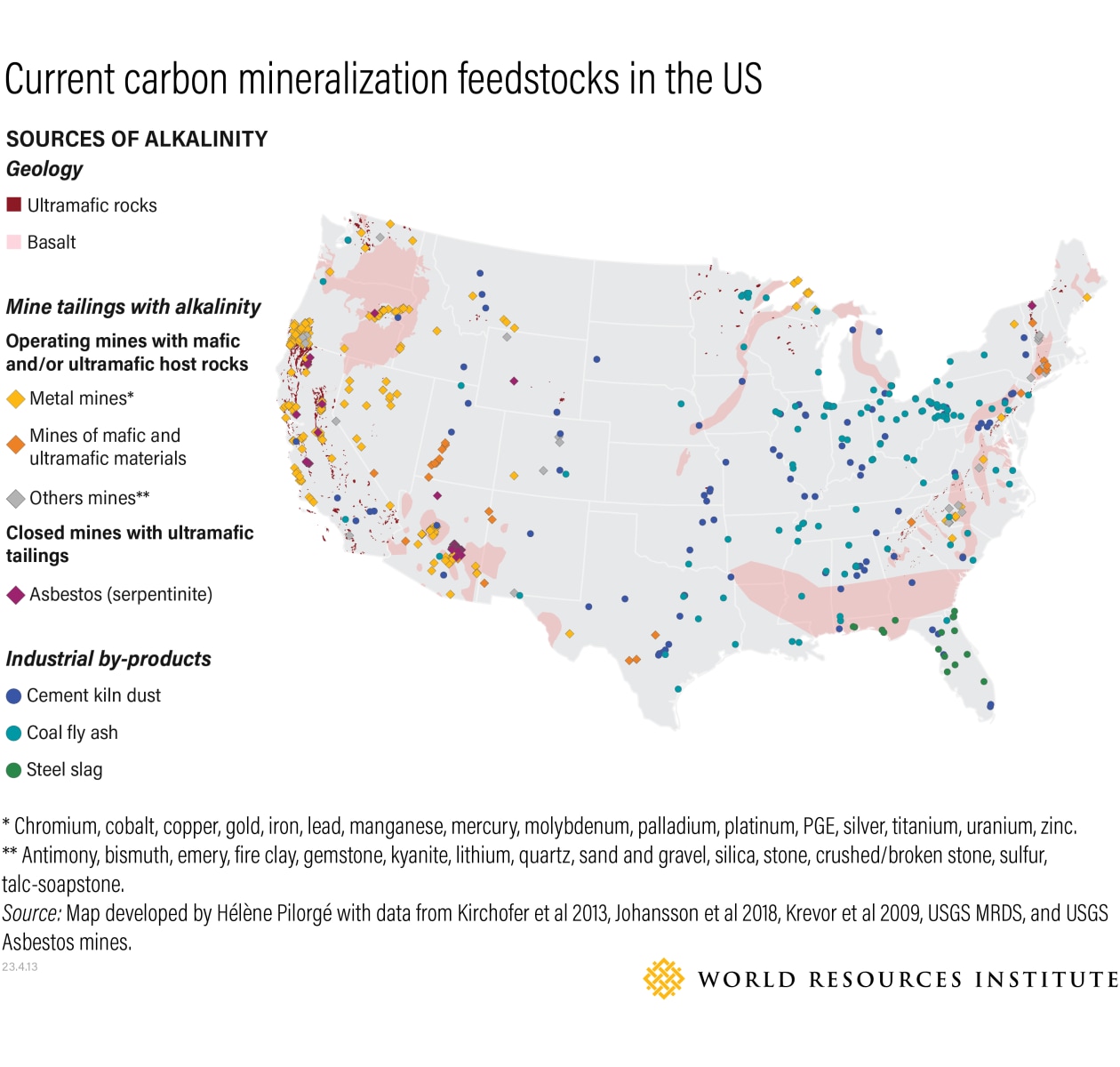
Surface
Surficial mineralization occurs when crushed alkaline feedstock, such as mined rocks, mine tailings or industrial waste, reacts with ambient or concentrated CO2. When crushed, alkaline rocks have a larger surface area, accelerating mineralization. Surficial mineralization can be done at industrial sites or mines that produce mine tailings as a by-product. If CO2 is captured on site, these alkaline feedstocks can be made to react with a more concentrated volume of CO2 at the same site, allowing for a quicker reaction than with ambient CO2.
Surficial mineralization also includes enhanced rock weathering, in which ultramafic or mafic rock is ground into powder and spread along coastlines or agricultural fields. Alkaline minerals within the rock powder react with ambient CO2, trapping it in solid carbonates. On coastlines, for example, the rock powder dissolves in seawater, converting dissolved CO2 in surface water into bicarbonates and carbonates, causing additional atmospheric CO2 to be absorbed into the ocean. This process acts as an antacid for the ocean, raising the water’s pH, which can reduce local ocean acidificationlocal ocean acidification.
Ex-situ mineralization occurs in high-pressure and/or high-temperature reactors where alkaline rocks react with concentrated CO2. Compared to other carbon mineralization pathways, ex-situ mineralization remains energy-intensive and costly. Efforts have therefore focused on using ex-situ approaches to produce value-added building materials, like concrete aggregate. Mineralization in concrete is attractive because it qualifies under the U.S. 45Q tax credit, an incentive for beneficial uses of captured CO2. It is estimated that globally between 0.1 and 1.4 gigatons of CO2 can be stored in concrete by 2050. Achieving net-negative emissions will, however, depend on the carbon-intensity of the concrete value chain.
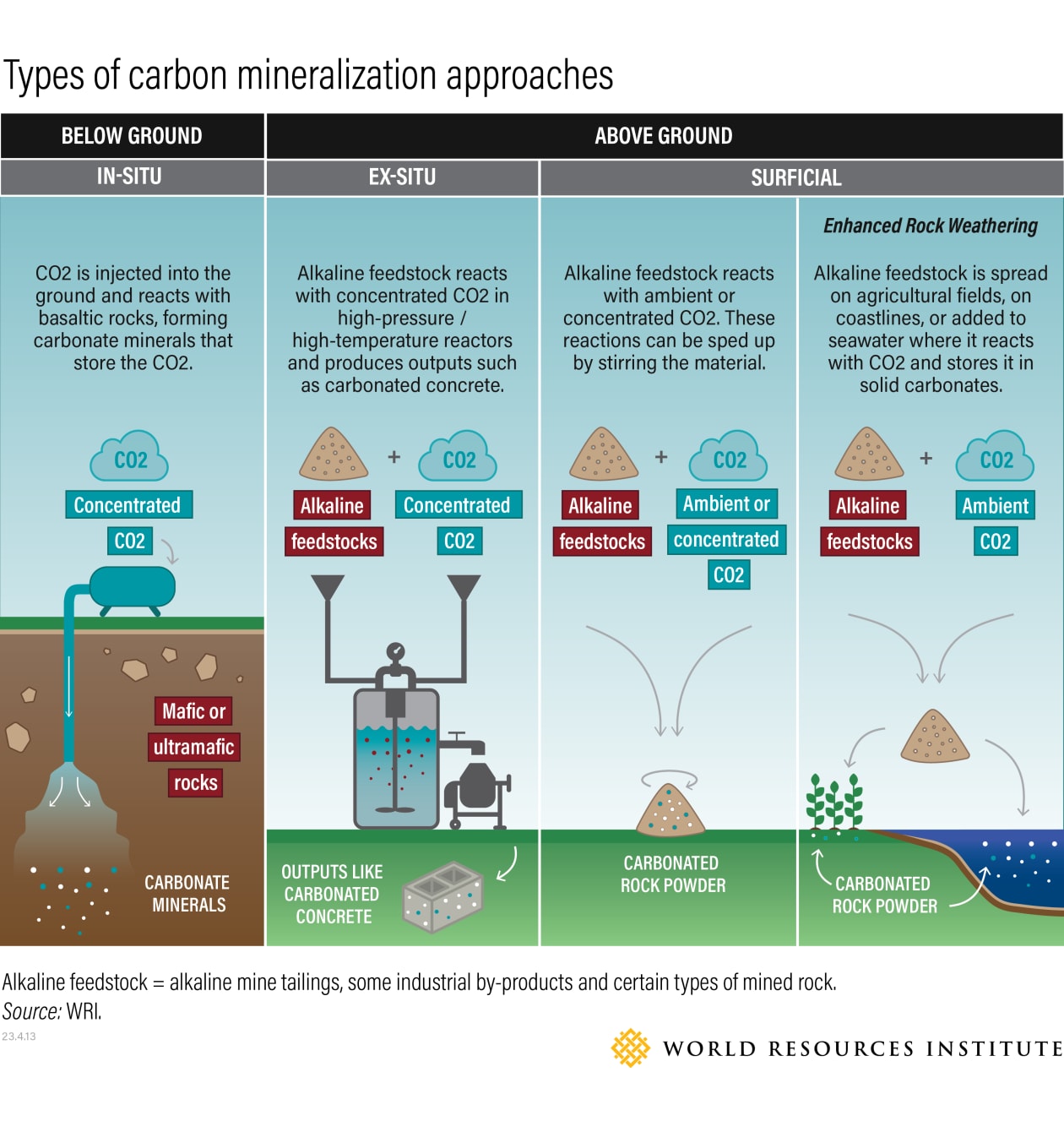
3) What are potential co-benefits of carbon mineralization?
Mineralization can be used to produce useful products. Some companies are already developing concrete blocks by injecting captured CO2 into the concrete before it hardens. This reaction creates calcium carbonate, which not only strengthens the concrete allowing for less cement to be used, but also permanently sequesters the CO2. Moreover, some mine tailings contain rare and valuable minerals like nickel or cobalt (needed for electric vehicle batteries) which can be extracted through indirect ex-situ mineralization. This can provide an additional revenue incentive that complies with the Biden administration’s goal of boosting domestic critical mineral production.
Mineralization could also help reduce some health hazards associated with some mine tailings. Asbestos tailings, for example, which are often stored at abandoned asbestos mines, are toxic when inhaled due to their fibrous crystal shape. When asbestos reacts with CO2 it forms magnesium carbonate minerals, sequestering CO2 and changing the shape of the crystal, which eliminates the health risk. However, techniques that use toxic mine tailings for mineralization must still be developed and tested at restricted sites and under strict oversight.
Another potential co-benefit is using alkaline rocks to reduce local ocean acidification and avoid subsequent damage to coral reefs and commercially valuable aquaculture. Rocks like olivine can be ground and spread on beaches or added directly to seawater where they react with dissolved CO2, forming solid carbonates that lock carbon away. More research and testing are needed to better understand how to maximize these co-benefits and how to minimize potential negative impacts on ecosystems.
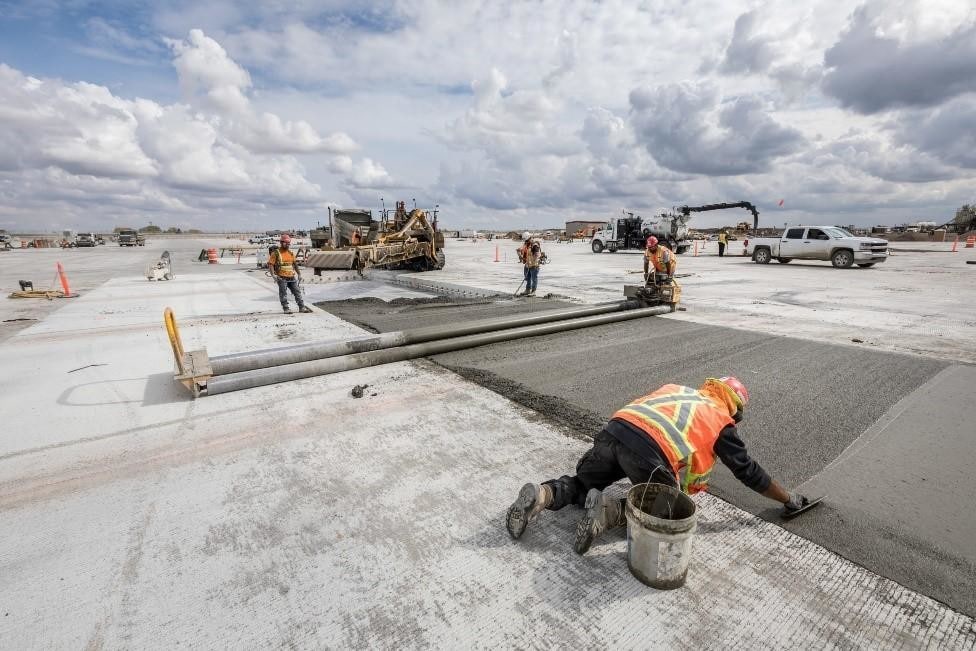
4) What are potential negative impacts of carbon mineralization?
Along with potential co-benefits, mineralization applications can also pose social and environmental risks that must be better understood and addressed before being deployed at scale.
Some approaches, such as enhanced rock weathering, may introduce metals such as nickel and chromium to the environment. These metals are toxic when accumulated and oxidized in soils or the ocean. In addition, storing carbonated mine tailings in tailing ponds can contaminate nearby surface and groundwater resources. To minimize this, some mine tailings can be pretreated to extract the heavy metals.
Although mineralization can help eliminate the health hazard associated with asbestos mine tailings, workers treating this waste can still be at risk of major health problems. Running fans to increase air flow over asbestos tailings can enhance mineralization, but also puts workers at risk of inhaling asbestos fibers. Therefore, strict precautionary measures are needed. Asbestos can, for example, be sprayed down with water to avoid the asbestos powder floating in the air.
What’s the World Economic Forum doing about climate change?
Some in-situ mineralization methods, such as the one applied by Carbfix in Iceland, require large amounts of water — about 25 tons per ton of CO2 injected. While recycling water is part of this process, total consumption could be an issue in water-scarce areas like the southwestern U.S. An alternative is for this method to be used offshore, using seawater that would avoid the scarcity issue. However, more energy would be required.
In addition to water resource concerns, induced local seismic activity needs to be considered. To minimize risks, regional seismicity tests must be regularly carried out and evaluated. Before CarbFix was injecting dissolved CO2, they were injecting wastewater into the basalt reservoir which significantly increased seismic activity. To address this issue, CO2 injection rates were adjusted, together with other preventative measures. As a result, seismic events decreased from 96 in 2011 to one in 2018, so that it now operates within regulatory boundaries. More data and further pilot projects are needed to better understand the associated risks of in-situ mineralization in basalts.
A key concern with scaling up carbon mineralization above ground is the need to increase mining to access large amounts of alkaline material, as well as grinding and transport — all of which require energy. Such increased mining would present negative social and environmental impacts associated with existing mining activities, such as water and air pollution and harm to human health.
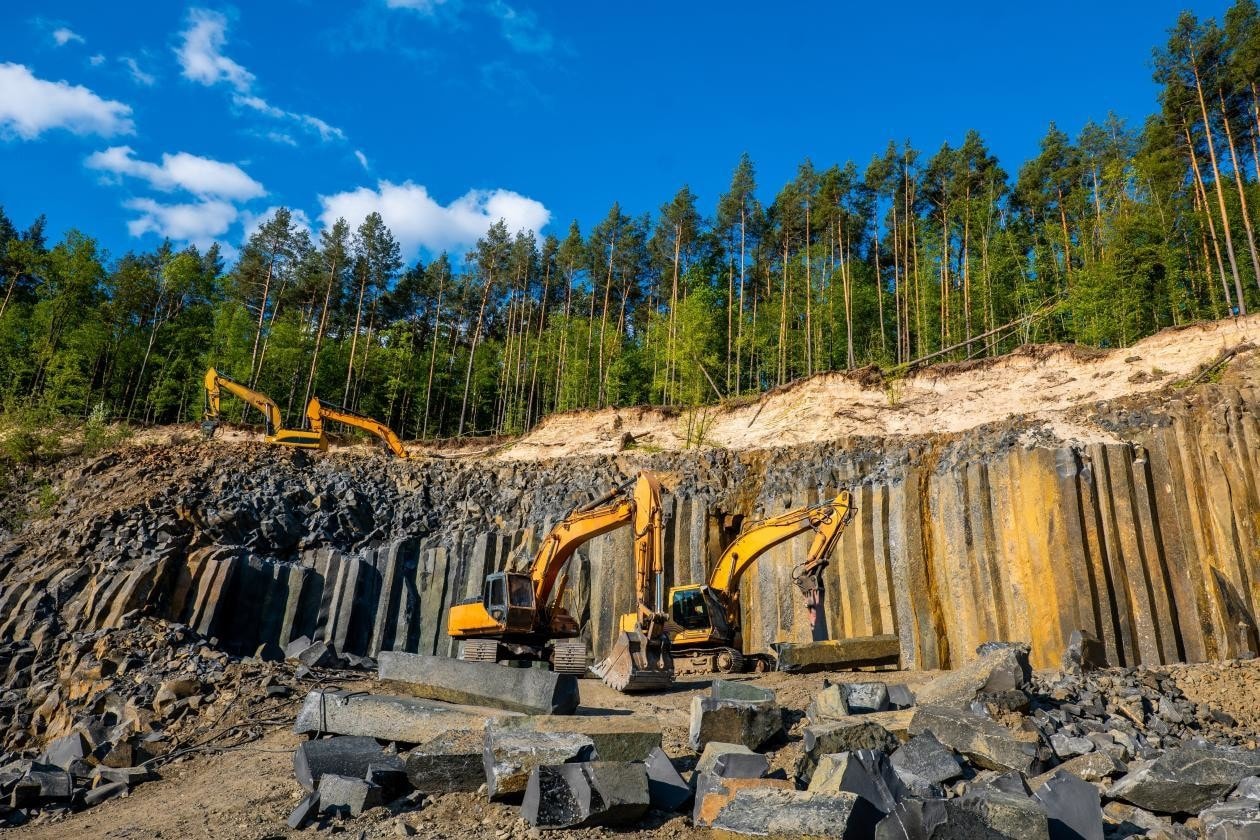
5) What’s needed to sustainably scale carbon mineralization?
Different mineralization pathways are at different stages of development. Subsurface mineralization in basalt formations is already happening in Iceland and the United States. Some surface mineralization approaches are also commercially available. To scale up carbon mineralization responsibly however, further research is needed to resolve scientific uncertainties and address risks. Standardized measurement and monitoring frameworks also need to be established.
Research needs
More pilot projects are needed to provide learnings from different sites and scales, allowing researchers to assess potential uncertainties and reduce risks. Despite the theoretical potential of removing large quantities of CO2, carbon mineralization may in part be constrained by logistical considerations, such as the transport of CO2 or feedstock. In addition, improved engineering and logistical practices are needed to minimize the emissions and costs associated with mineralization approaches.
In addition, further study of the chemistry of certain minerals and how they respond to CO2 mineralization treatments, as well as overall rock characteristics, is needed. Datasets are often not standardized, making it challenging to compare data on rock composition, grain size, pressure, porosity, permeability and surface area. More data will also be needed on potential feedstock quantities in different locations, as well as their concentrations and compositions.
Updated, high-resolution mapping of where suitable rocks and minerals are located is needed at more comprehensive scales. For example, existing USGS data on operating and closed mines has not been updated in a decade. It is crucial to have current information that can be integrated into comprehensive databases to better identify the location, amount, chemical and mineral composition of waste rocks and mine tailings and estimate their CO2 storage potential. More collaboration with industry will be necessary to better map and understand the amounts of alkaline waste available for CO2 mineralization, as well as data on the asbestos content of certain ultramafic rocks.
Improved and standardized measurement and monitoring tools
Standardized methods and high-quality MRV (measuring, reporting and verification) protocols must be developed and applied across projects to accurately measure how much CO2 has been removed by a project over a particular timeframe. Currently, methods are not yet standardized and companies are applying their own unique protocols, making it difficult to compare across projects.
Carbon removed through surficial mineralization, for example, is challenging to account for and monitor because oceans, coasts and soils, where mine tailings and crushed rocks are spread, are open systems (as compared to a closed-system DAC plant). Observing the amount of carbon removed through subsurface mineralization also has its challenges, as it cannot be observed directly, and the collection of physical samples can be complicated and expensive. CO2 mineralized and stored through ex-situ mineralization is more easily monitored, especially when stored in products such as cement. High-quality MRV systems are needed to guarantee consistency and integrity across projects to ensure their positive climate impact.
Developing mineralization as a climate solution
Carbon mineralization presents significant potential as a carbon removal approach, within a larger suite of carbon removal and climate actions, to help reach global climate goals. For carbon mineralization to be considered a key CDR pathway, research and pilot projects will have to increase. Uncertainties around where and how to conduct mineralization projects, as well as the potential negative environmental and social impacts of projects, must be minimized. Standardized and holistic MRV protocols are also needed to ensure that carbon mineralization is scaled up responsibly.
Don't miss any update on this topic
Create a free account and access your personalized content collection with our latest publications and analyses.
License and Republishing
World Economic Forum articles may be republished in accordance with the Creative Commons Attribution-NonCommercial-NoDerivatives 4.0 International Public License, and in accordance with our Terms of Use.
The views expressed in this article are those of the author alone and not the World Economic Forum.
Related topics:
The Agenda Weekly
A weekly update of the most important issues driving the global agenda
You can unsubscribe at any time using the link in our emails. For more details, review our privacy policy.
More on Climate ActionSee all
Madeleine North
July 4, 2024
Nasim Pour
July 3, 2024
Luis Alvarado
July 3, 2024
Cristen Hemingway Jaynes
July 2, 2024
Cristen Hemingway Jaynes
July 2, 2024