Seawater electrolysis: a hydrogen revolution or technological dud? Here are the numbers
One method to produce green hydrogen, an important part of the net-zero transition, is seawater electrolysis — but do the numbers add up? Image: REUTERS/Mike Blake
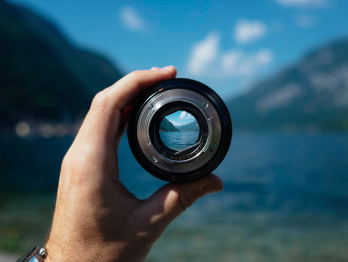
Get involved with our crowdsourced digital platform to deliver impact at scale
Stay up to date:
Tech and Innovation
Listen to the article
- Seawater electrolysis has been hailed as a wonder tech for creating green hydrogen — seen by some as the fuel of the future.
- Others have argued that seawater electrolysis is simply not viable when you dig into the stats.
- To get to the bottom of it, we need to dig into the figures.
The advent of the hydrogen economy in the last four or so years, have ignited numerous old ideas to be revisited.
Some of these ideas include plasma electrolysis and seawater electrolysis — in which water is broken down into its constituent parts through electrolysis — both of which have been explored in the past and decried as either not economically or technologically viable.
Media coverage, meanwhile, has described seawater electrolysis as the next big technological breakthrough and an enabler of the hydrogen economy, painting it as a near-miracle level breakthrough. But this is inaccurate: seawater electrolysis is a solution that is looking for a problem.
A large portion of the vast hydrogen investments end up supporting technological breakthroughs that promised more efficient, cheaper and more reliable hydrogen production pathways. However, many proponents of these technological breakthroughs are entirely newcomers to the hydrogen industry. They often do not have suitable backgrounds to understand these concepts in sufficient detail — not to mention that many have never done electrochemistry before.
Understanding how we do electrolysis today
Traditionally, water used for electrolysis undergoes purification through a commercial reverse osmosis process and may require subsequent deionization to remove remaining ions. Water quality supplied to the majority of modern water electrolysis systems must comply with ASTM Type II standard — essentially a cleaner quality of water — although many producers recommended compliance with ASTM Type I, the cleanest standard of commercially useable water.
ASTM Type I feedstock quality can easily be achieved by commercial reverse osmosis and deionisation plants. In essence, a vast number of large hydrogen projects announced globally will likely rely on seawater feedstock, however, they will require this extra processing step prior to feeding pure water into electrolyser to produce hydrogen.
State-of-the-art, commercially available and broadly deployed reverse osmosis plus deionization is today's standard for seawater treatment and purification.
The question is whether skipping this water purification step to electrolyse seawater directly is a better approach. To understand whether we should adopt the seawater approach, where we move directly to seawater electrolysis, or keep current approaches in which we use reverse osmosis to purify water, requires us to delve into the numbers.
Seawater electrolysis: the numbers
Neither the hydrogen economy nor water electrolysis are new concepts. Water electrolysers have been in use for over a century and their performance and materials have evolved significantly over that period, resulting in robust, efficient and sophisticated solutions today. The same applies to water treatment solutions.
Let’s start with exploring the energy consumption of modern water electrolysis and water purification systems. To produce 1 kg of hydrogen, 8.92 kgs of water is needed. In reality, we need more because of losses — some water does not end up as hydrogen and oxygen and leaves the system unprocessed. For the purpose of calculations, an average water consumption of 11 l/kg of hydrogen was assumed.
In perfect conditions, production of 1 kg of hydrogen requires 39.4 kWh/kg. In reality, and due to various inefficiencies, water electrolysis consumes an average of 50 – 55 kWh/kg. Energy requirement of modern water treatment plants depends largely on quality of feedstock water, quality of permeate and type of technology used. Most commonly used reverse osmosis plants consume roughly 0.0012 kWh/l and 0.0046 kWh/l for brackish water and seawater feedstock, respectively. In addition, depending on the quality of produced water, a demineralized water treatment plant might be deployed to further purify the permeate.
The energy consumption of a demineralized water treatment plant is in the range of 0.0016 kWh/l. As such, energy consumption to purify the seawater to meet the feedstock purity requirements for electrolyser ranges roughly from 0.055 to 0.077 kWh/kg H2 (given assumed water consumption of 11 l/kg of hydrogen) or less than 0.2% of the total energy consumption to produce hydrogen.
The energy benefits of seawater electrolysis are negligible, and so are the cost benefits.
Modern reverse and demineralization plants are very cost-effective. As reported by Hydrogen Europe, the total cost for water desalination is around 0.85 USD/m3, which then adds about 0.0075 USD/kg to the production cost of hydrogen.
Instead of seawater electrolysis, future electrolyser developments operating on ultrapure water should focus on optimizing parameters such as cost expenditure and energy efficiency. An improvement of only 0.2% in energy consumption would theoretically give the same results as operating electrolysers with seawater.
Due to osmotic pressure increase and entropy decrease, electrolysis on seawater will require more energy compared to electrolysis on ultrapure water, so any potential savings in energy will even out by the laws of thermodynamics.
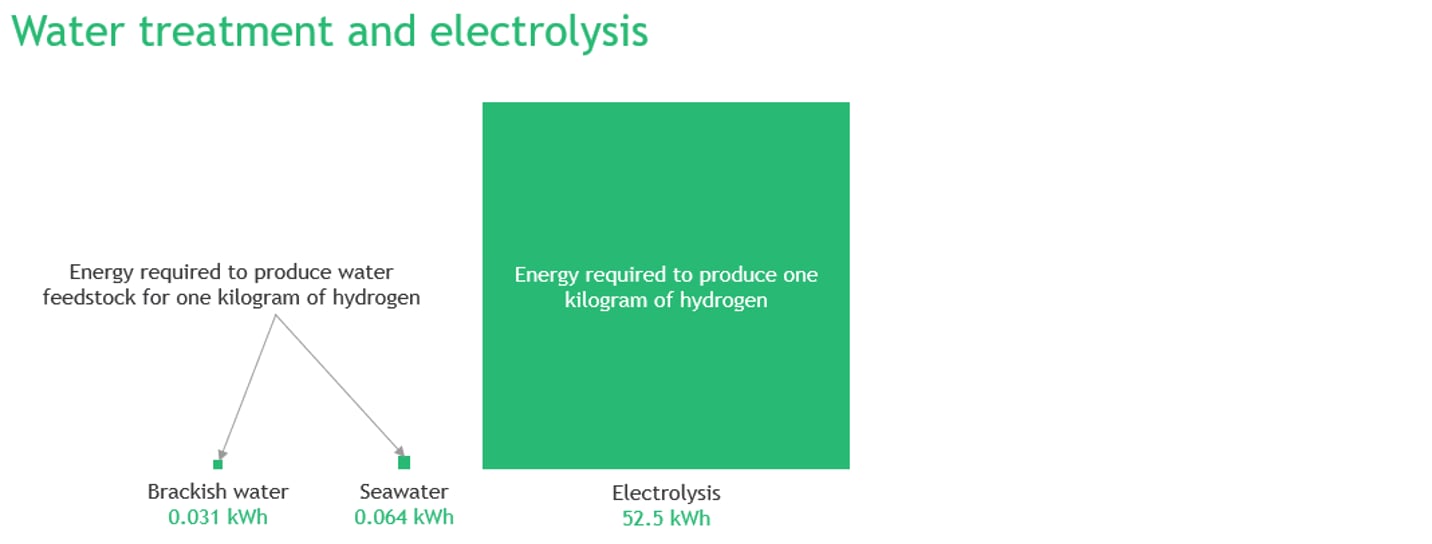
Electrolysis on seawater — a dead end?
The complexity of performing electrochemistry in seawater must not be overlooked.
Seawater contains a large number of organic and inorganic species, including microbes and metal ions. Biofouling is going to become a large issue in seawater electrolysis, while metals ions, many of which have lower redox potential than 1.8 V, will be over time over-electrodeposited onto the electrodes effectively reducing the electrocatalytic performance of hydrogen production.
Various species present in seawater may also decompose, resulting in contamination of produced hydrogen (and oxygen). For example, and as rightfully pointed out by Service, corrosive and toxic chlorine gas can evolve during seawater electrolysis.
Finally, there are good reasons that a major industrial process used globally to convert highly concentrated sodium chloride brine into lye, chlorine gas and hydrogen uses ultrapure water and pure sodium chloride instead of relying on high sodium chloride concentrations contained in the seawater.
Asking the right questions
The hydrogen economy still has a multitude of high-impact challenges that require their own unique solutions. Some of these may include enabling electrolyser manufacturing at scale or automating electrolyser manufacturing processes to reduce capital expenditure of electrolyser systems.
Instead of allocating vast amounts of funding towards impractical solutions or solutions where the challenge overrides the benefits, let’s focus the efforts on solving problems with high impact and clear benefits, filling in these missing gaps within the hydrogen and clean fuels value chains.
Direct seawater electrolysis would result in decreased electrolyser durability, higher energy and cost expenditure and lower purity for produced hydrogen. Even thermodynamics are unfavourable of this direct pathway.
Don't miss any update on this topic
Create a free account and access your personalized content collection with our latest publications and analyses.
License and Republishing
World Economic Forum articles may be republished in accordance with the Creative Commons Attribution-NonCommercial-NoDerivatives 4.0 International Public License, and in accordance with our Terms of Use.
The views expressed in this article are those of the author alone and not the World Economic Forum.
The Agenda Weekly
A weekly update of the most important issues driving the global agenda
You can unsubscribe at any time using the link in our emails. For more details, review our privacy policy.
More on Emerging TechnologiesSee all
Eleni Kemene, Bart Valkhof and Thapelo Tladi
July 22, 2024
Henry Ajder
July 19, 2024
Sebastian Buckup
July 18, 2024
Nii Simmonds and Ayodele Okeowo
July 17, 2024
Jerome Desbonnet and Oded Vanunu
July 16, 2024
Tariq Malik and Prerna Saxena
July 12, 2024