Bringing clean energy closer
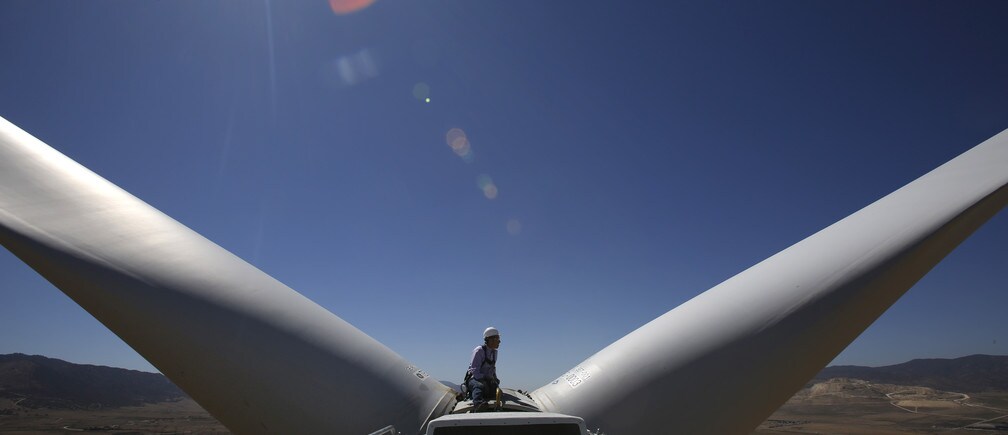
Solid oxide fuel cells and solid oxide electrolysis cells hold the promise of highly efficient energy conversion, with lower pollution, to meet increasing global energy demands. But these devices need good catalysis to speed up the oxygen reduction reaction (ORR), either at the cathode in a fuel cell or at the anode in an electrolysis cell.
“This is really the bottleneck of these two clean energy techniques,” says MIT materials science and engineering graduate student Qiyang Lu.
Lu is working with fellow nuclear science and engineering graduate student Lixin Sun under Associate Professor of Nuclear Science and Engineering Bilge Yildiz to develop techniques for applying strain to materials to accelerate oxygen-reduction reactions for application in solid-oxide fuel cells. Lu conducts the experimental side of the research, focusing on oxygen exchange on the surface of cathode materials, while Sun explores the computational side, studying ionic activity of solid-state electrolytes and cathodes.
“The motivation is to lower the operating temperature of solid oxide fuel cells; however, both the ion diffusion and surface reactions are thermally activated. But you need a certain temperature to maintain high efficiency. So what we can do is use lattice strain to enhance materials properties, for example, accelerate the cathode reactions or oxygen diffusion in the electrolyte to maintain the same conversion efficiency at the lower temperature. That’s the final goal that we are fighting for,” Sun says.
Lu studies neodymium nickel oxide, or neodymium nickelate, Nd2NiO4+δ, where δ signifies the amount of excess oxygens in the material, and the higher the δ (delta) is, the better the performance of this material in a fuel cell is. “It’s a complex oxide with two different cations, and oxygen as an anion, and this has very promising electrochemical properties, which can be used as the solid oxide fuel cathode material,” Lu says.
Harnessing elastic strain
It is known that stretching or compressing an oxide or ceramic material changes some of its properties. “What we want to find out is when you stretch this kind of oxide, can the catalytic property of this oxide be enhanced by strain?” Lu explains.
There are two kinds of strain: elastic strain, the kind you see in a stretched rubber band, where the material will return to its initial state when the strain is relaxed, and plastic strain, where the material is deformed such that it can’t return to its original state, Sun says. This latter type of strain is accommodated by forming dislocations in the material.
Lu and coworkers are studying how elastic strain can make the oxide perform better as cathode material for future solid-oxide fuel cells. Lu synthesizes the strained nanoscale thin films at MIT using a technique called pulsed laser deposition. He does experimental characterization of his strained films at Brookhaven National Lab and Lawrence Berkeley National Lab, in particular using in situ X-ray photoelectron spectroscopy (XPS), and at MIT by a combination of X-ray diffraction, ex situ XPS, and scanning probe techniques.
Sun works on fluorite-structured doped oxides (like ceria and zirconia) and focuses on enhancing ionic activity in the electrolyte material. Sun reviewed the scientific literature on strain effects and found that results are widely inconsistent, ranging from eight orders of magnitude enhancement in a sandwich-structured YSZ/STO thin film with a fluorite crystal lattice to no enhancement at all. “There is a large scattering among the experimental results in the literature,” Sun says. “There must be an explanation.” Accounting for dislocations Sun says her examination of prior studies found that some of the materials studied contained dislocations, which are extended defects in the microstructure of the material, which would relax the strain. “A dislocation means a discontinuity of the crystal structure that is induced by mechanical deformation or during the crystal growth,” says Sun. In a perfect bulk-crystal structure, atoms are well-aligned in a lattice, Sun explains. However, when strain leads to plastic deformation, the crystal structure undergoes a discontinuity by forming a dislocation. “Around this defect, all the other atoms still follow the same crystal structure, but they are displaced from their perfect positions.”
A high density of dislocations could explain why different researchers are getting different results in studies of the performance of these thin films, Sun suggests. But direct experimental study of the dislocation can be elusive because the defects can have a radius as small as 5 angstroms, or just five-tenths of a nanometer, and it is very difficult to isolate the effect of one dislocation at a time. “That’s why you need the computational simulation, to treat the problem atomistically and one dislocation at a time,” Sun says. She uses ab initio, molecular dynamics, and Monte Carlo calculations to understand the influence of the dislocation from the atomic scale, modeling oxide ion conductivity in doped ceria-based oxide material.
“In the solid oxide fuel cell, you need to move the oxygen from one side to the other. For electrolyte, you want that oxygen to be moved as fast as and as much as possible,” Sun explains.
While some researchers have argued that dislocations can speed up oxygen transfer, others argue that dislocation can relax the elastic strain in a material and reduce the ionic conductivity.
“So all we want to do is clarify this problem, whether the dislocation can accelerate or slow down the ionic conductivity, and based on that, give guidance for the design of electrolyte structures,” Sun explains.
Highway analogy
Sun likens the oxygen transport to cars on a highway: When you drive a car onto an uncrowded highway, you can drive very fast, but if everyone gets onto the highway at once, you get a traffic jam. Sun speculates that the same thing happens with oxygen transport in doped fluorite oxides, like ceria and zirconia. A few defects may speed up the process, but if many defects get into close proximity of the dislocation this may cause a slowdown.
Yildiz says the finding in the oxide material was unexpected based on prior studies of dislocation in metals. “When you come to the oxides that we use in fuel cells, electrolyzers, membranes, that have intrinsically fast ion conduction, this concept of ‘freeway dislocations’ needs reconsideration, because such oxides have charged defects and the electrostatic interactions among charged defects may lead to trapping of oxygen,” she explains. “Such strong electrostatic interactions do not occur in metals. The fast-path assumption adopted directly from metals to oxides may not work for the design of oxide microstructures.”
Sun says her research sheds a new light on the prior large volume of inconclusive experimental studies. “We think this work is important because we are dealing with structures that appear in the real material that we are going to use in the technological application, and knowing the role of dislocations can help to design the microstructure of the material for better performance,” Sun says.
Third-year graduate student Sun received her bachelor’s degree in physics from Peking University in China; third-year graduate student Lu received his BS in materials science and engineering at Tshingua University in China.
Published in collaboration with MIT News
Author: Denis Paiste is a science writer at the Massachusetts Institute of Technology Materials Processing Center.
Image: Wind turbine at a wind farm in Tehachapi, California. REUTERS/Mario Anzuoni
Don't miss any update on this topic
Create a free account and access your personalized content collection with our latest publications and analyses.
License and Republishing
World Economic Forum articles may be republished in accordance with the Creative Commons Attribution-NonCommercial-NoDerivatives 4.0 International Public License, and in accordance with our Terms of Use.
The views expressed in this article are those of the author alone and not the World Economic Forum.
Stay up to date:
Innovation
Related topics:
The Agenda Weekly
A weekly update of the most important issues driving the global agenda
You can unsubscribe at any time using the link in our emails. For more details, review our privacy policy.