How fluorescent proteins are lighting up science
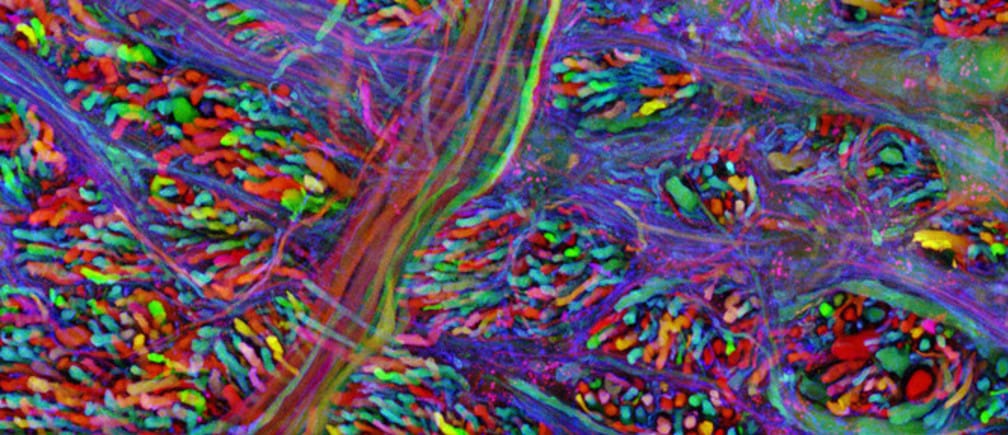
Stay up to date:
Fourth Industrial Revolution
When you look up at the blue sky, where are the stars that you see at night? They’re there but we can’t see them. A firefly flitting across a field is invisible to us during the day, but at night we can easily spot its flashes. Similarly, proteins, viruses, parasites and bacteria inside living cells can’t be seen by the naked eye under normal conditions. But a technique using a fluorescent protein can light up cells’ molecular machinations like a microscopic flashlight.
The first fluorescent protein found in nature comes from the crystal jellyfish, Aequorea victoria, where it is responsible for the green light emitted by its photo organs. It’s called green fluorescent protein (GFP). We don’t know why these jellyfish have this lit-up feature.
Fluorescent proteins absorb light with short wavelengths, such as blue light, and immediately return it with a different color light that has a longer wavelength, such as green. In Aequorea victoria, a protein named aequorin produces blue light which GFP converts into the green light emitted by the jellyfish’s photo organs. This visibility under standard conditions is extremely rare; most other organisms have fluorescent proteins that are only visible if they are illuminated by external blue light sources.
After the green fluorescent jellyfish protein, many other fluorescent proteins have been both found in nature and created in the lab. We now have a spectrum of fluorescent colors available to us that make previously invisible biological structures and processes visible in blazing fluorescent glory. Many new applications reliant on these colors are being published on a regular basis.

Paul Steinbach and Roger Y. Tsien, University of California, San Diego, CC BY-SA
Shining a light on imaging
Fluorescent protein technology has led to many other interesting developments designed to improve imaging with these glowing molecules.
CaMPARI is one new technique, short for calcium-modulated photoactivatable ratiometric integrator. By exploiting the fact that calcium concentrations change when nerve cells send signals, CaMPARI is able to light up all the neurons that have fired in a living organism. The technique is based on a fluorescent protein called EOS, which changes its fluorescence from green to red. In fruit flies, zebrafish and mice, CaMPARI-genetically-modified neurons fluoresce red if they are active and green if they are less active.

Looger Lab (HHMI/Janelia), Science, VOL 347, ISSUE 6223.
Before CaMPARI, all the fluorescent calcium indicators available temporarily lit up when the neuron fired. They couldn’t record the firing history of neurons or indicate whether a neuron had fired in the past. According to Loren Looger, one of the researchers who worked on the development of CaMPARI, “The most enabling thing about this technology may be that you don’t have to have your organism under a microscope during your experiment. So we can now visualize neural activity in fly larvae crawling on a plate or fish swimming in a dish.”
Expanding and transparent brains
Even with the help of light emitted by fluorescent proteins, it’s difficult to image neurons tangled deep within the brain. Ed Boyden, a neuroscientist from MIT, has created a method to expand brains to make fluorescent neurons deep within the brain more visible. He uses acrylate, which forms a dense mesh to hold the brain in place and expand in the presence of water thereby inflating the brain equally by about 4.5 times in each direction. It’s a lot like a diaper expanding when it gets wet. Boyden thinks that this “expansion microscopy may provide a key tool for comprehensive, precise, circuit-wide, brain mapping.”

The Deisseroth Lab
One of the reasons expansion microscopy is so useful is that the brain can be made see-through before it is blown up several sizes larger. In 2013 Karl Deisseroth and Viviana Gradinaru at Stanford published a method called CLARITY that removes opaque molecules such as fats and makes the brain transparent without changing its shape. According to Thomas Insel, director of the US National Institute of Mental Health, “This is probably one of the most important advances for doing neuroanatomy in decades.” Since developing CLARITY for brains, Gradinaru has extended the method to all other organs including an entire mouse.
Both of these methods can be applied to brains that have been genetically modified with fluorescent proteins, therefore allowing for the visualization of neurons deep within the brain.

Wellcome Images, CC BY-NC-ND
In 2008, the three scientists responsible for taking GFP from the jellyfish and making it a common tool used in over a million experiments all over the world were awarded the 100th Nobel Prize in chemistry. And in 2014 three other scientists were awarded the Nobel Prize for using fluorescent protein to increase the resolution of light microscopes.
Revolutionary and resilient
I’ve been researching the photochemistry and photophysics of fluorescent proteins since they were first used in imaging technology in 1994, I’ve written two books on them, and still I’m stunned by the many different ways in which this fairly simple protein can be used. Perhaps I shouldn’t be surprised that plasmid DNA molecules coding for GFP have survived space flight – not inside the rocket, but on the outside where they were exposed to 1800F (1000C) temperatures and mad friction. 53% of the DNA intentionally placed inside the screw heads in the TEXUS-49 rocket mission expressed fully fluorescent GFP when inserted into cells upon return to earth.
Like stars at night, fluorescent proteins have been lighting up science for the last 20 years. And it won’t be long before they’re guiding surgeons to tumorous growths during surgery and allowing researchers to switch on and off selected biomolecular processes.
This article is published in collaboration with The Conversation. Publication does not imply endorsement of views by the World Economic Forum.
To keep up with the Agenda subscribe to our weekly newsletter.
Author: Marc Zimmer Professor of Chemistry and Dean of Studies at Connecticut College
Don't miss any update on this topic
Create a free account and access your personalized content collection with our latest publications and analyses.
License and Republishing
World Economic Forum articles may be republished in accordance with the Creative Commons Attribution-NonCommercial-NoDerivatives 4.0 International Public License, and in accordance with our Terms of Use.
The views expressed in this article are those of the author alone and not the World Economic Forum.
Related topics:
Forum Stories newsletter
Bringing you weekly curated insights and analysis on the global issues that matter.
More on Emerging TechnologiesSee all
Robert Opp and Keyzom Ngodup Massally
April 4, 2025
Ibrahim Abdullah Alshunaifi and Caroline Tasse
April 3, 2025
David Elliott
April 2, 2025