How biotechnology is evolving in the Fourth Industrial Revolution
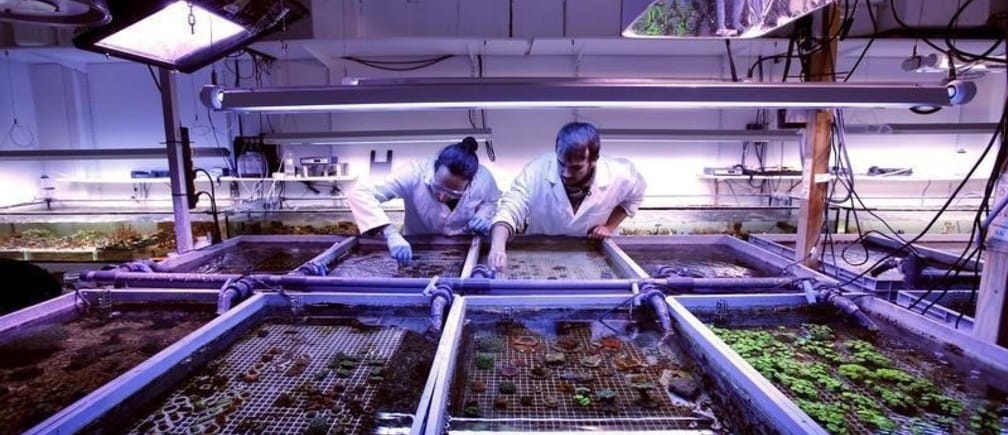
Modern biotechnology could mitigate humans' impact on the planet Image: REUTERS/Jean-Paul Pelissier
Victor de Lorenzo
Head, Molecular Environmental Microbiology Laboratory, Spanish Council for Scientific Research (CSIC)When modern biotechnology emerged in the late 1970s, it was first applied in the health sector, with the onset of recombinant DNA. One decade later, the same molecular approaches reached the agricultural and food industries, not without controversy. Finally, biotechnologists started to enrich the capabilities of industrial microbial processes by bringing new genes to live catalysts and modifying their genomes to fit pre-specified production needs. Though such operations were deemed as genetic engineering, in reality the engineering aspects were more metaphor than reality. Instead, what we might call genetic bricolage (i.e. trial-and-error) dominated the field quite successfully for a long time.
However, the arrival of systems biology by the end of the 1990s, and the emergence of synthetic biology in the early 2000s, completely changed the game of designing microorganisms, and even higher living systems, as agents for industrial-scale transformations of feedstocks of diverse origins into valuable products. Microorganisms capable of producing a variety of chemicals of industrial importance, including dicarboxylic acids (succinic acid and adipic acid), diols (1,3-propanediol and 1,4-butanediol), diamines (putrescine and cadaverine) and many others have been developed. Some bacteria and yeast long known by the industry can now be genetically reprogrammed and repurposed, for example to produce lipids serving as biofuel precursors. Even non-natural chemicals such as gasoline and terephthalic acid can now be produced by metabolic engineering.
Furthermore, contemporary biotechnology has produced biomaterials including polysaccharides (microbial cellulose), proteins (spider silk), and even formerly synthetic polymers (polylactate and poly[lactate-co-glycolate]) by fermentation of engineered microorganisms. Some strains have been successfully designed to produce polyhydroxyalkanoates, a family of diverse biopolyesters, for applications in environmentally friendly packaging, medicine and smart materials.
Moreover, innovative bioprocessing is increasingly developed based on the unusual properties of extant biological systems (e.g. extremophiles) to run fermentations, for example in seawater and non-sterile conditions. Not in vain, systems-guided metabolic engineering was selected by the WEF as one of 2016’s top 10 emerging technologies.
However, despite these successes, the chemical and manufacturing industries are still largely reluctant to adopt bio-based transformations and bio-inspired practices that could take over many extant oil-based processes. The limiting factor is the difficulty of converting laboratory-scale operations into economically viable, industrially-sized equivalents. The instability of live catalysts, the consumption of large volumes of water and difficulties in downstream processing have deterred what in many cases would otherwise be a welcome transition in the methods of production.
So is advanced biotech restricted to producing just small amounts of high value-added molecules? To overcome the impasse for the Fourth Industrial Revolution, various issues need to be addressed, both on the biological and industrial sides. Genomic and phenotypic stability of live catalysts is paramount for matching the efficiency of bioprocesses to those already existing in the purely chemical realm. This is not just a technical problem, but a fundamental scientific question that needs to be addressed. The interplay of stress versus chromosome constancy (including implanted genetic devices) under production conditions must be investigated at large, and new approaches for its control developed.
In the meantime, cell-free production systems may offer an interim solution to the challenge of predictability. Additional biological questions such as gene expression under non-saturating water conditions, physiology at very high cell densities and minimization of cell debris due to spontaneous lysis will surely help to make bioprocesses more appealing to big industry.
But the challenges are not only biological. They are also found on the process engineering side. In contrast with the spectacular advances of recent years in genomic editing, basic fermentation methods have remained largely the same since the ancient Egyptians produced beer. An aqueous nutrient medium in a pot or barrel is inoculated with an active agent and left to evolve until the desired transformation occurs, or until the desired compounds are generated.
Modern engineering has been able to control fermentation to an extraordinary degree, and has certainly extended bioreactor types towards much more sophisticated designs. But most industrial bioproduction still relies on vessels filled with a considerable volume of water-based, sterile media inoculated with a single monoculture of the adequate strain. Productivity is then measured in grammes per litre.
There is ample room for improving this state of affairs, by encouraging investigation into how some natural systems produce compounds in large amounts. For instance, couldn’t we get inspiration from the awesome productivity of cows’ udders or rubber trees, as we design a different type of bioreactor with very little water, sterile functioning and easy operation? What is the engineering logic that makes these systems so efficient?
There is much to learn from such optimally evolved biological reactors, beyond just trying to adapt biological catalysts to already existing platforms. Production at the site of need or at the point of care also demands new concepts for generation of biomolecules in miniaturized, portable settings, that will surely differ from what we have now.
Finally, modern biotechnology has much to offer for overcoming the alienation between the global industrial metabolism and the geobiochemical cycles of the biosphere. Waste from unchecked industrial, agricultural and urban development has generated large amounts of greenhouse gases, non-degradable plastics, micropollutants, major unbalances of nitrogen and phosphorus, and an unmanageable volume of lignocellulosic residues.
The global environmental microbiome could become our main ally for reconnecting such anthropogenic waste with the cycling of elements that operates our planet. If a few chemical reactions (e.g. the Haber-Bosch process for nitrogen fixation) were able to change the ecology of our planet, we may also explore ways of mitigating our impact by deploying large-scale bio-based interventions aimed at restoring former environmental balances and creating new ones.
Of course, this would not be a mere academic experiment but a multi-tiered mission involving many stakeholders and requiring careful governance. Yet, in view of the phenomenal environmental challenges that the next generation faces, inaction and business-as-usual surely pose the highest risk.
Don't miss any update on this topic
Create a free account and access your personalized content collection with our latest publications and analyses.
License and Republishing
World Economic Forum articles may be republished in accordance with the Creative Commons Attribution-NonCommercial-NoDerivatives 4.0 International Public License, and in accordance with our Terms of Use.
The views expressed in this article are those of the author alone and not the World Economic Forum.
Stay up to date:
Biotechnology
Forum Stories newsletter
Bringing you weekly curated insights and analysis on the global issues that matter.