Scientists just got closer to making nuclear fusion work
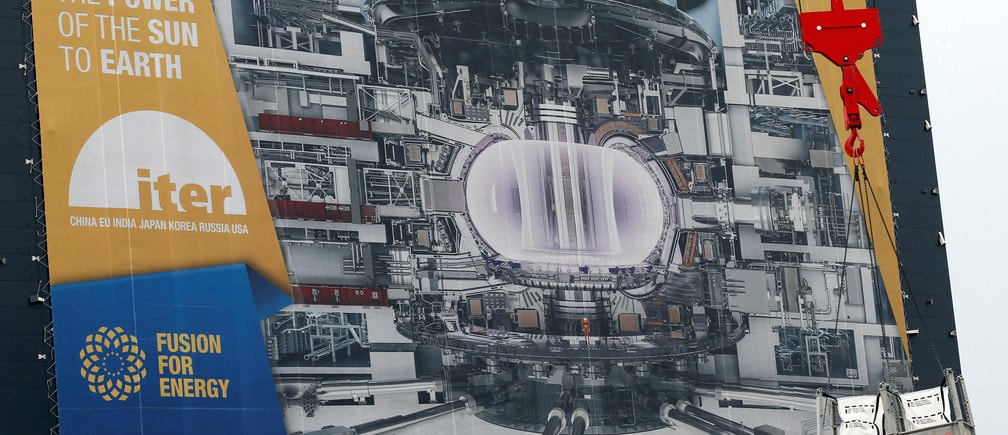
Nuclear fusion is the holy grail of unlimited, zero-carbon energy. Image: REUTERS/Jean-Paul Pelissier
Proponents of nuclear fusion see it is as a clean and virtually limitless energy source that could power the future. But while researchers are confident they can make it work, realizing the long-held dream of fusion power is proving far from easy.
Potentially offering an inexhaustible supply of zero-carbon energy, nuclear fusion has shown great promise for decades but is yet to be viable at scale because maintaining a fusion reaction requires more power than it generates.
However, recent advances in the quest for fusion power have reignited hopes that it can be made feasible.
Scientists in China have built a fusion reactor that in November became the first in the world to reach 100 million degrees Celsius. That’s nearly seven times hotter than the sun’s core and the temperature at which hydrogen atoms can begin to fuse into helium.
The achievement by China’s Institute of Plasma Physics at its Experimental Advanced Superconducting Tokamak (EAST) is a milestone on the fusion journey, and will provide valuable insights for the International Thermonuclear Experimental Reactor (ITER) project, a collaboration between the European Union, India, Japan, China, Russia, South Korea and the United States.
At an estimated cost of $25 billion, the consortium is building a prototype fusion reactor, called a tokamak, in southern France. It aims to conduct a first test of super-heated plasma by 2025 and generate first full-power fusion by 2035.
Although the ITER is the biggest and most expensive project, there are more than a dozen other fusion research initiatives under way.
Last year a privately funded UK venture called Tokamak Energy announced its plasma had hit 15 million degrees Celsius for the first time.
A collaboration between MIT and the start-up Commonwealth Fusion Systems is designing a fusion reactor capable of producing more power than it consumes. Their research will complement the work done by ITER.
And the Canadian government announced last year it is investing US$37.5 million in General Fusion, a company founded in 2002 that focuses on an approach known as magnetized target fusion.
What is 'fusion' exactly?
Fusion is the reaction that powers the Sun. It’s produced when two light atoms fuse into one under extreme pressure and temperature. The total mass of the new atom is less than that of the two that formed it; the "missing" mass is given off as energy, as described by Albert Einstein's equation E=mc2.
Fission, which is the energy source in current nuclear power stations, involves splitting an atom’s nucleus.
Fusion has the potential to deliver much more power than fission, but without the long-lasting radioactive waste.
There are several "recipes" for cooking up fusion, which rely on different atomic combinations.
The most promising combination for power on Earth today is the fusion of a deuterium atom with a tritium one. The process, which requires temperatures of approximately 39 million degrees Celsius, produces 17.6 million electron volts of energy.
Deuterium is a promising ingredient because it is an isotope of hydrogen. In turn, hydrogen is a key part of water. A gallon of seawater (3.8 litres) could produce as much energy as 300 gallons (1,136 litres) of petrol.
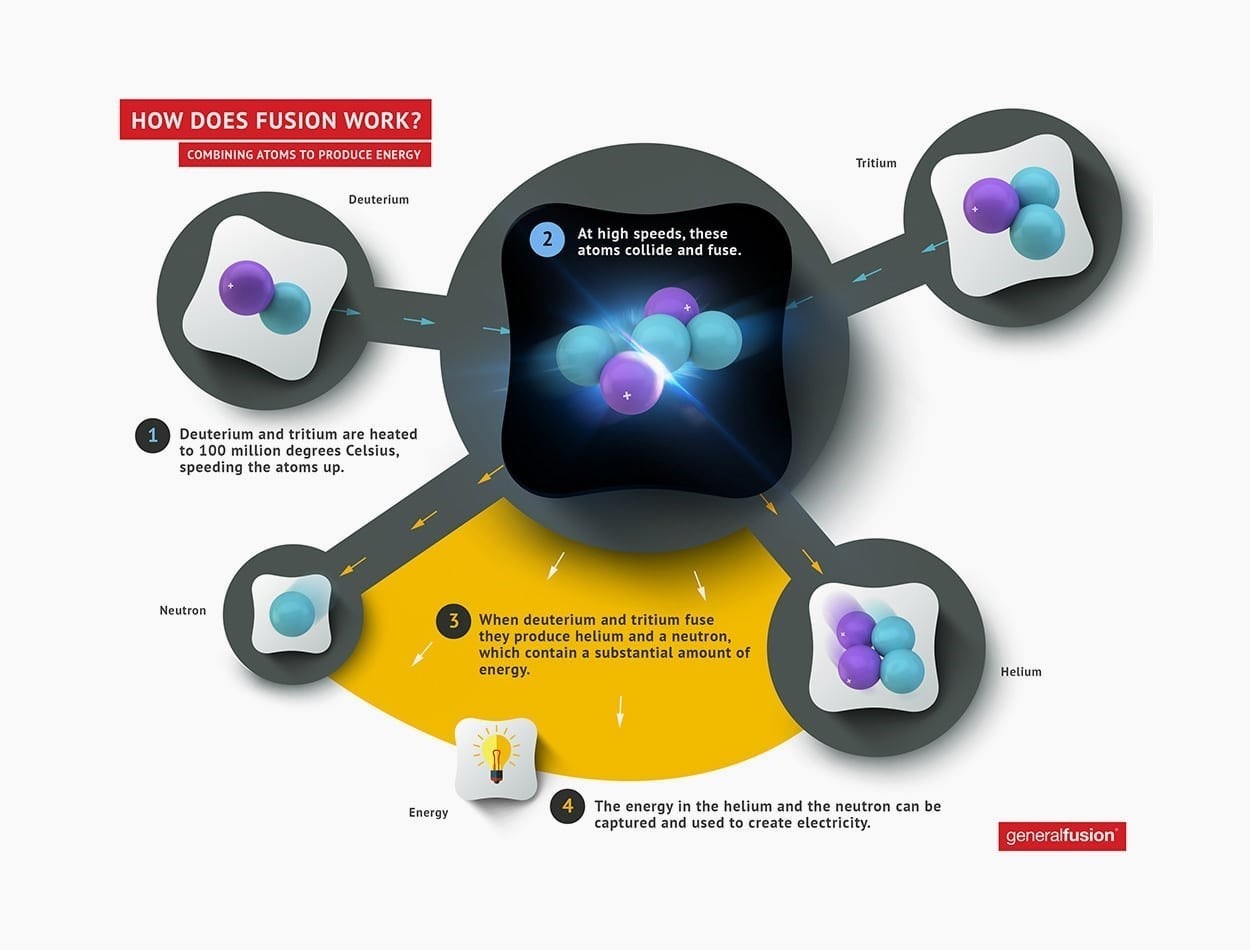
Putting theory into practice
While fusion power offers the prospect of a clean source of energy, it has also presented many so-far-insurmountable scientific and engineering challenges.
In the sun, massive gravitational forces create the right conditions for fusion in its core, but on Earth they are much harder to achieve.
Fusion fuel – different isotopes of hydrogen – must be heated to extreme temperatures, and must be kept stable under intense pressure, and dense enough and confined for long enough to allow the nuclei to fuse.
And this is where progress has been made. Advances in magnet technology have enabled researchers at MIT to propose a new design for a practical compact fusion reactor that might deliver a net power output perhaps within the next decade or so.
New superconducting magnets would enable the reactor to operate in a sustained way, producing a steady power output, unlike today’s experimental reactors that can only operate for a few seconds at a time without overheating.
The era of practical fusion power may finally be coming nearer.
Don't miss any update on this topic
Create a free account and access your personalized content collection with our latest publications and analyses.
License and Republishing
World Economic Forum articles may be republished in accordance with the Creative Commons Attribution-NonCommercial-NoDerivatives 4.0 International Public License, and in accordance with our Terms of Use.
The views expressed in this article are those of the author alone and not the World Economic Forum.
Stay up to date:
Energy Transition
The Agenda Weekly
A weekly update of the most important issues driving the global agenda
You can unsubscribe at any time using the link in our emails. For more details, review our privacy policy.
More on Energy TransitionSee all
Miranda Barker
November 29, 2024